Ancient Upheavals Show How to Geoengineer a Stable Climate
The current ice age is a geologically rare event, threatened by human activity. Emissions reduction won’t be enough to resolve climate change. Instead, we must learn from the ancient past to stabilize
This article by Patrick Mellor was originally published on Palladium Magazine on January 28, 2019. It was featured in PALLADIUM 07: Garden Planet.
In April of last year, the concentration of carbon dioxide in the Earth’s atmosphere reached 410 ppm. This is the highest in over 3 million years, and much higher than the pre-industrial average of 280 ppm. The last time CO2 levels were this high, in the middle Pliocene epoch, the average global temperature was around 3 °C greater than today, and sea levels were 25 meters higher. The time before that, in the middle Miocene, sea level was 40-50 meters higher.
Assuming “business as usual,” CO2 concentration is projected to be 600 to 800 ppm by the end of the century. Optimistic projections not involving sustained negative emissions starting at mid-century give an average of ~2°C warming by 2100. Even that is considered by authorities to be a problem, but it’s less than the paleoclimatic analog.
It’s usually assumed that if we reduce emissions, then CO2 will steadily decline on its own due to natural sequestration processes. This is based on the notion of a set residence time in the atmosphere—essentially a guaranteed life cycle which these kinds of predictions can be based on—as if the pre-industrial climate was some kind of strong equilibrium to which excursions will quickly return.
But that’s not really how it works. In fact, the assumption that our pre-industrial climate was a robust equilibrium is an existentially dangerous one. If there’s more carbon around, the biosphere will just operate with more carbon in the cycle, including in the air, until some process causes permanent sequestration. To understand this, as well as the unusual and metastable nature of our current climate, we have to go back to the geological history of climate change.
The Current Climate Is An Icy Anomaly
Prior to 20 million years ago, both carbon dioxide concentration and temperature were significantly above current levels, and had remained there for most of earth’s history.
Even during the much of the cooling trend of the last 20 million years, the Arctic Ocean was ice free in the summer, and the northern margins of the surrounding continents, where today there is barren tundra, were clothed in temperate forest. The Antarctic Ice Sheet covered only a small area around the pole, the rest of the continent supporting mixed forests of conifers and southern beeches. The coastlines of the continents were many miles further inland than today.
In contrast to the usual apocalyptic narratives, the tropics were not scorched wastelands. They were covered with luxuriant rainforests containing up to 100 species of apes, including our own ancestors. Temperatures were up to 10 ℃ higher than today at the poles, but the tropics were not much hotter. Recent observations are showing that warming is concentrated in high latitudes, causing mostly reduced seasonal variations.
Prior to the last 20 million years, the climate was mostly warm and wet, with much reduced seasonality.
The last time the climate was anything like today—in other words, the last time there was an ice age, the current one being the Quaternary glaciation—was the late Carboniferous period. That was 300 million years ago, before the dinosaurs, with an ice age lasting approximately 60 million years. Prior to that, the only other ice age since the advent of multicellular life was the Ordovician glaciation, about 450 million years ago, at which time the atmosphere had not yet reached its modern composition.
The key point to understand is that the recent low carbon, low temperature condition is a significant anomaly from the norm. We are in an interglacial period—with reduced but still significant glaciation—of an ice age which has lasted for the past 2.6 million years. Ice ages are rare: the Earth has had significant polar ice caps for at most 20% of the last 540 million years.
The remaining 80%, including the entire Mesozoic era, saw what are often described as hothouse or greenhouse conditions. The greenhouse state is the default equilibrium. Glacial or icehouse periods, on the other hand, are historically not stable. They are comparatively short term events occurring because of particular contingent conditions.
Most of Earth’s history has been warm, wet, and high-carbon. Ice ages like the current one are rare, few, and short. The recent climate has not been a long-term equilibrium, but a short-term metastable condition. This is key background for any discussion of global warming, and any possible response.
Prehistoric Volcanic Warming
Though there are other factors, historical large changes in the climate equilibrium are closely correlated with addition and removal of CO2 from the atmosphere. The addition is usually due to massive and sustained volcanic activity on a scale unseen for tens of millions of years. For example, large areas of Siberia and Northern India are covered with the remains of lava flows extending hundreds of miles, from eruptions which continued for tens of millenia, known as flood basalts or traps.
The Siberian Traps were formed simultaneously with the Permian-Triassic mass extinction, the worst in history, where 95% of marine and 70% of terrestrial species were wiped out. Coming close on the heels of this extinction event, the Wrangellia eruptions in the Carnian stage of the early Triassic caused a further mass injection of CO2 into the atmosphere, and another episode of sudden warming. This increased evaporation from the oceans to such an extent that extreme precipitation caused almost continuous flooding across the entire planet. Current evidence suggests that average global rainfall reached the levels now seen in temperate rainforests on the Pacific Northwest, for a period of 2 million years. Known as the Carnian Pluvial Event, this caused another large scale turnover in the biosphere, instrumental in the evolution of the dinosaurs.
The Indian flows, or Deccan Traps, happened at the same time as the extinction of the dinosaurs, and were possibly caused or intensified by the asteroid impact that caused the extinction. This caused a warming period leading to the Paleocene-Eocene Thermal Maximum, an event much studied as a possible analogue of current climate change.
The volcanic hotspot underlying Yellowstone National Park is a possible source of a future flood basalt event.
Historically, these eruptions seem to cause an initial global cooling, caused by the release of sun-blocking aerosols like SO2 into the upper atmosphere, and then a large degree of warming caused by CO2 as the Sulfur dissipates. The associated mass extinctions are likely exacerbated by this climate whiplash. They also prolong the effects: the initial event and dimmed cooling period reduces biological productivity, reducing the ability of the biosphere to sequester carbon. This exacerbates ocean acidification as CO2 dissolves into the water. Ocean acidification, combined with the sudden reheating, in turn keeps biological productivity much reduced and slows recovery. It can then take millions of years for the biosphere to return the CO2 level to an equilibrium.
In contrast, mass extraction of CO2 from the atmosphere is associated with events where unusual factors enable the removal of large quantities of plant material from circulation before the biomass either decomposes or is consumed by animals (which would release the CO2 absorbed by plant life back into the atmosphere). One example of this would be mass burial. Once icehouse conditions are established—that is, ice age conditions with relatively lower temperatures, such as the present day—they are maintained until the sequestration mechanism ceases to operate.
In the early history of the Earth, this happened multiple times, and these ice ages are termed Snowball Earth events. The earliest one was likely caused by cyanobacteria, the first widely successful photosynthetic organism. The advent of photosynthesis both removed CO2 directly, and removed methane, another potent greenhouse gas, by oxidation. The transition to an oxidizing atmospheric chemistry caused a mass extinction, as other life wasn’t adapted to a harsh oxygen atmosphere. As a result, the cyanobacteria could draw down CO2 until nearly the entire surface of the planet was covered in ice. These events may have ended by the injection of CO2 through eruption of flood basalts through the ice cover.
The important point to take away from this is that massive CO2 addition to the atmosphere has historically been caused by volcanic eruptions, and slow carbon drawdown has occurred through the response of the biosphere to this addition, returning conditions to a hothouse equilibrium. Large-scale CO2 removal is caused by distinctive and contingent biological events, rather than by any kind of ongoing and stable geophysical process, and results in a metastable icehouse state.
Our current addition of CO2 differs from this pattern by being caused by industrial activity, and occurring on a far shorter timescale and higher rate than is typical, although with a lower potential total addition.
Contextualizing Anthropogenic Warming
For comparison to our own activity, the most intense phase of the Deccan Traps eruptions, 66 million years ago, released several tens of thousands of gigatons of CO2 into the atmosphere, over a period of about 30,000 years. Since 1750, we have emitted about 1500 gigatons, half of this in the last 40 years. We have roughly 3000 gigatons of potential emissions from easily accessible fossil fuels remaining in the ground, although to release this we would have to extract and burn all the world’s coal, which is unlikely. So, even assuming these figures to be underestimates, and factoring in CO2 equivalents in methane and other warming gases, total realized and potential anthropogenic emissions are unlikely to exceed 4000 gigatons.
This is dwarfed by the effect of the largest Deccan eruptions. Realistically, we will only ever emit about a tenth of the CO2 released in a flood basalt volcanic event; we will probably not use all available coal reserves as the carbon intensity of economic growth is either flat or on a slowly declining trend. The major difference is that our emissions are occurring over a period of centuries, rather than tens of millennia. Even the most extreme natural events would release only on the order of 1 gigaton per year, whereas in 2017, global CO2 emissions were about 41 gigatons.
So our own maximum total impact is well within the limits of the largest natural events that have occurred in prehistory, but is happening much faster, and is therefore significant. Judging by these prehistorical analogues, by default we can expect a very large impact: a mass extinction, significant loss of coastal land, and large changes to global weather patterns.
Most importantly, the unprecedented rate of our emissions may cause significant instability in the climate, which is already precariously balanced in an unusual ice age. Such instability has been seen recently in the transition from the last glacial maximum to the current interglacial period, though this was not directly caused by CO2 emissions.
This transition was not gradual or stable. Temperatures rose and fell multiple degrees within decades. In the Younger Dryas event, only 13,000 years ago, global temperatures declined 2-6℃ from values similar to the present, within about 30 years, after having risen on a similar timescale. The colder climate lasted 1000 years, causing glaciers to advance once more across Eurasia and North America, and then ended as abruptly as it started. There is evidence that the sudden increase in aridity caused during this time disrupted and postponed early development of agriculture in the Levant.
Our economic systems and patterns of settlement are not equipped to deal well with this degree of instability nor its possible result of a return to the hothouse equilibrium.
Analogs For Intervention
It’s obvious that something will need to be done about this, either to adapt to changing global climate patterns, or ideally to control them. Fortunately, the geological record does not only forecast harsh change, but also contains example mechanisms of mass carbon removal from the atmosphere, which could help us to understand our options.
The two most relevant massive drawdown events occur during the Carboniferous period, and the Eocene epoch:
In the Carboniferous, around 300 million years ago over a period of tens of millions of years, CO2 concentration declined tenfold from over 2000 ppm to about 200 ppm, similar to levels at the most recent glacial maximum, and the only other time they have fallen this low in the last 500 million years. Unsurprisingly, this also resulted in the previously mentioned ice age, a particularly long and deep one. The carbon drawdown was caused by the evolution of land plants before terrestrial herbivores and fungi became adept at digesting wood. Huge forests proliferated across the continents and dead trees simply laid where they fell without decomposing efficiently, until they were eventually buried.
Millions of years of this process sequestered enough carbon to raise the oxygen concentration of the atmosphere to 35% (today it is 20%). This allowed the evolution of giant insects, whose respiratory system depended on this elevated oxygen, and terrifyingly destructive firestorms, where even wet wood would burn. When fungi, termites, and herbivorous vertebrates evolved and proliferated enough to end the sequestration process, and start returning carbon trapped in dead vegetation to the atmosphere, the ice age ended. Glaciation did not occur on Earth for another 270 million years. Much of the coal we burn now represents carbon removed from the atmosphere during this ice age.
The second example, the Azolla event, was only discovered in the last 20 years, and was extremely specific in its mechanism. In the early Eocene, global surface temperatures were 8 ℃ higher than today. Tropical forests extended almost to the poles, and atmospheric CO2 concentration was 3500 ppm, in contrast to today’s 400 ppm. Around 49 million years ago, the global climate suddenly cooled by 6 ℃, with a simultaneous drop of atmospheric CO2 to 650 ppm. The cause of this drop in temperature and CO2 was unknown until a convincing hypothesis was proposed in 2004:
The Arctic Coring Expedition took advantage of reduced ice cover to take core samples from the Lomonosov Ridge, in the Northern Arctic Ocean. To their surprise, they discovered huge deposits of the freshwater aquatic fern Azolla locked under the seabed. These deposits, up to 20 meters deep, were laid down 49 million years ago, sustained over 800,000 years, and exactly coinciding with the observed drop in temperature and CO2. Noting this correlation, the researchers proposed a causal link, now widely accepted, between the strange growth of Azolla and the massive drawdown in atmospheric carbon dioxide, accounting for the sudden global cooling in the Eocene.
Azolla is incapable of growth in salt water, and so was only able to grow in huge volumes in the Arctic Ocean due to a set of unusual factors involving a freshwater surface layer. These factors also ensured that the carbon absorbed by the fern through photosynthesis was sequestered on the seabed rather than being returned to the atmosphere when the plants decomposed.
During the Eocene, the Arctic Ocean had limited water exchange with the rest of the world oceans, and received the flow of a number of large rivers. The lower density freshwater from these rivers pooled on the surface, causing a low salinity layer to form. This layer prevented mixing of the water column, and created a seabed with extremely low oxygen levels, while also enabling Azolla to proliferate across the surface. As a floating plant with leaves above the water, Azolla had direct access to atmospheric CO2. Additionally, and unusually among ferns, Azolla is able to fix nitrogen directly from the atmosphere using a bacterial symbiosis, removing a further limitation to growth. Most decomposing organisms require oxygen for their metabolism, and this was severely depleted on the seabed. Therefore, when the ferns died and sank, they were preserved intact until buried by sediment, and the carbon they had absorbed in life was locked into the sedimentary rock under the sea. Ironically, much of the oil and natural gas resources currently under active exploration in the Arctic are composed of these Azolla deposits.
A common and vital lesson can be drawn from these historic events: previous episodes of warming have only ended when specific events in the biosphere, like the Carboniferous drawdown and the Azolla event, caused mass drawdown of CO2. The cessation of emissions alone, and the background rate of carbon extraction from the atmosphere, have never been sufficient to reduce atmospheric CO2 either to a level or on a timescale relevant to human civilization.
Possible Geoengineering Methods
Even currently locked-in emissions and warming may cause dramatic climate instability, or a phase transition to a stable hothouse equilibrium, where the factors maintaining the equilibrium may be powerful enough to resist any attempt to return. Therefore, active intervention in the climate system, not merely changing structure of economic activity, seems necessary to avoid the potential of an uncontrolled climate catastrophe.
Such intervention could be no different in principle to the examples we’ve just looked at. The Azolla event is particularly informative, as it shows how specific conditions obtaining in the physical environment can cause a self-sustaining biological process with a favorable ratio of carbon extraction to energy investment.
In any case, some kind of geoengineering or controlled carbon drawdown is necessary to ensure optimal and stable conditions for human civilization.
While previous examples of biological carbon drawdown have mostly occurred over timescales of at minimum several millennia, any human effort would need to be effective on a much shorter timescale.
There are four realistic candidate geoengineering methods that could be used to solve this problem. These are direct air capture (DAC), bioenergy with carbon capture and storage (BECCS), injection of aerosols into the high atmosphere, and oceanic iron fertilization:
Direct Air Capture is simple, but is currently too expensive at approximately $100 per ton of CO2. DAC startups don’t even plan to sequester, but rather to produce carbon-neutral fuels for profit, which isn’t helpful for geoengineering. Government funding could provide incentive for sequestration, but the expense would be too high, and the technology won’t be mature enough to scale any time soon; Canadian startup Carbon Engineering’s pilot plant only extracts one ton per day.
BECCS consists of biomass being cultivated at large scale and used as feedstock to create biofuels such as ethanol, replacing fossil fuels in the same roles, or directly burned to generate electrical power. The CO2 released in the process is captured by scrubber systems and injected deep underground, usually into porous rock formations. This enables a portion of the CO2 collected by the plants in photosynthesis to be sequestered, while the rest is used to create carbon neutral biofuel and electricity. In theory, this enables a BECCS system to achieve net negative emissions while remaining economically viable from the sale of biofuels and electrical power.
BECCS is an elaboration of simple carbon capture and storage, where scrubbers are used to capture exhaust gases from fossil fuel burning, or biomass is pyrolyzed into biochar to be buried or used as soil amendment. These methods are already in operation where carbon credits act as an economic incentive.
Because of its close integration with existing technologies and emissions sources, BECCS is currently the favored method for achieving net negative emissions, but like DAC suffers from problems of scalability. A BECCS project would need to economically support both its fuel and power production, and its method of carbon sequestration, using the sale of the commodities produced. This is unlikely to be viable in the near term without either significant carbon taxes or increases in fossil fuel prices, which the advent of fracking has made unlikely for now.
Using current methods, removing 10 gigatons of CO2 annually using BECCS would require a land area the size of India to be brought under cultivation for this purpose alone, which would entail an expansion of agricultural land without compare in recent history and be ecologically disastrous in itself. If BECCS projects replaced existing agriculture, this would displace the cultivation of food on arable land. BECCS will almost certainly be a component of future strategies to effect carbon drawdown, but cannot be rolled out on the scale required within a short time frame, and would be too destructive as a sole solution.
Atmospheric Aerosol Injection is the next method. It is possible to provide a direct cooling effect by injecting aerosols—most models using SO2—into the stratosphere to partially block out the sun. This method is not included in mainstream roadmaps, but would be extremely economical and deceptively efficient. By directly reducing incoming solar radiation and causing global dimming, SO2 injection mimics the short term effects of a large volcanic eruption, and enables the prevention of warming without addressing CO2 emissions at all. By this mechanism, aerosol pollution from industry likely masked some of the impact of increasing CO2 levels during the 20th century.
While SO2 causes acid rain if released at low altitude, if injected into the upper atmosphere it would have little to no effect at ground level, and would provide effective cooling if continuously replenished. SO2 could be injected through large hoses attached to tethered balloons, from pressurized canisters aboard specialized or commercial aircraft, or through airburst artillery. Addition of a few million tons of SO2 yearly would be sufficient to offset the warming effect of a doubled CO2 concentration. This is a comparatively small quantity, and could be distributed by a fleet of modified 747 airliners for a cost of 1 or 2 billion dollars yearly. This would provide effective mitigation of climate change within months. With costs comparable to those of operating a small airline, this method could be implemented by most states, or even by wealthy individuals.
The ease of implementation of this method makes it almost inevitable that it will at some point be employed unilaterally. For example, if a strong heat wave occurs in a city in Southern Asia during a period of high humidity, as will likely happen at some point in the next few decades, and wet bulb temperatures rise above 35℃, this will cause mass casualties directly attributable to climate change. Currently, the impacts of heatwaves are seen as an increase in the background death rate, and mainly affect the very old, very young, and people with existing health problems. But in this case, previously healthy people will start dying in the streets without access to air conditioning.
This level of obvious impact will create an imperative for the government of the country concerned to do something, anything, to mitigate the situation. Given the low cost of aerosol injection, and its immediate effects, there is a strong possibility that injecting SO2 into the stratosphere will be their response, with easy justification using the argument that the tragedy was the result of centuries of emissions by large wealthy nations. This could have complex geopolitical consequences, given that aerosol injection will modify the global climate quickly and obviously, causing easily observable whitening of the sky.
At first glance, aerosol injection seems to be the ultimate “get out of jail free card” for climate change, and it does have a place in preventing immediate catastrophic consequences of runaway warming. I would not be at all surprised if it were employed at some point. It does not, however, provide a long term solution.
First of all, shading the planet will not affect the rate of ocean acidification, which creates severe problems in its own right. Dissolution of CO2 into the ocean lowers the pH of seawater, disrupting the oceanic biosphere. We can see this happening already: since 1750, the average pH of the world ocean has decreased from 8.25 to 8.14.
Second, any aerosol injection system requires continuous management and calibration to give the right degree of cooling. Successful short term management of climate using this method will take the pressure off and thus reduce the employment of more long term solutions that address CO2 directly. If CO2 concentrations continue to increase during a period of effective solar radiation management, an unstable situation could result where any interruption or miscalibration in aerosol injection will result in sudden extreme warming. This is the same mechanism that caused previous mass extinctions after flood basalt events, as we noted above.
Oceanic Iron Fertilization. The ideal geoengineering method for tackling the carbon problem would mimic a naturally occurring process which has been shown to mitigate past episodes of global warming, would not be prohibitively expensive or environmentally destructive, would have beneficial effects beyond those of reducing climate change, would not create dependency on continuous careful management, and would have self-limiting negative consequences if misapplied. Such a method exists, has been demonstrated experimentally for decades, and does not require the creation of any massive infrastructure or technological innovation. This method is oceanic iron fertilization.
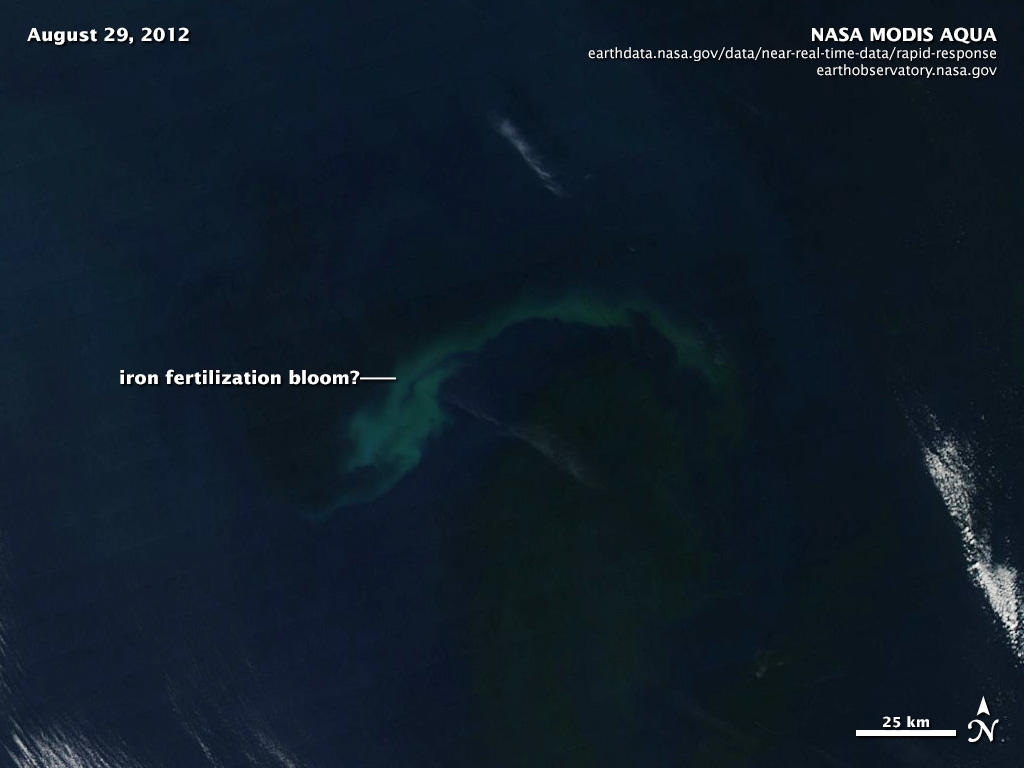
Iron fertilization takes advantage of the fact that the growth of marine phytoplankton is primarily limited by micronutrient availability, specifically that of iron, and that repletion of available iron in the photic zone of the oceans is able to induce massive algal blooms, which then sink into the abyss, sequestering vast quantities of carbon. Iron fertilization has been experimentally demonstrated twelve times since 1993, most notably in 2007 in the South Atlantic by an Indo-German consortium (project LOHAFEX, 2007), and in the North Pacific in 2012, by the Haida Salmon Restoration Corporation (HSRC). In both cases the addition of ferrous sulfate in quantities between 10 and 100 tons resulted in massive plankton blooms visible from space, with an estimated production of up to 100,000 tons of algae per ton of added iron. Even assuming that much of the carbon contained in this algae is eventually returned to the atmosphere, this is a most favorable ratio. Oceanographer John Martin was aware of this as early as 1988, when he stated “give me half a tanker of iron, and I will give you an ice age.”
Several iron fertilization experiments have been of doubtful legal status, and societal reactions to them have been polarized both in the popular press and academic contexts. The HSRC release was organized by the HSRC in collaboration with Russ George, founder of the San Francisco based company Planktos Inc.. They were careful to release the iron in technically international waters, which gives an idea of the ambiguous legal situation. George was subsequently described in much of the media as a “rogue geoengineer” and “Pacific Ocean hacker,” and accused of manipulating the Haida into supporting his scheme. In reality the project was collaborative and the Haida were equal partners, standing to benefit economically from the expected increase in salmon catch.
As intended, there was a correlated massive increase of the 2013 year class of sockeye salmon by up to 400% due to increased food availability. The causality is still debatable, but a similar increase in salmon population occurred in the years following the 2008 eruption of the Kasatochi volcano in Alaska, which introduced a large quantity of iron-rich dust into the atmosphere, providing strong evidence that iron fertilization in general increases fish stocks.
Currently, the majority of iron input to the deep oceans originates from desert dust storms over the Sahara and Central Australia, which also contribute to the fertility of the Amazon rainforest, due to their global transport by wind currents in the high atmosphere. There is evidence that the increased input of windblown iron rich dust into the oceans due to aridity in the last glacial maximum was partly responsible for maintaining low CO2 and cold temperatures. The quantity of this windblown dust has been decreasing in recent decades, and this is correlated with a decline in ocean fertility.
Another major source of iron and other nutrients is whale feces. Whales distribute nutrients throughout the world ocean by feeding on krill and small fish in productive regions of upwelling, and spreading nutrients throughout their migrations by defecating in surface waters. Krill contain so much iron that the feces of whales become red, containing 10 million times the concentration of iron in ocean surface waters. But the global population of large whales is still only at most 20-30% of that before industrial whaling, despite a decades old ban. Studies of average water transparency suggest that there has been an annual decline of 1% in ocean phytoplankton populations throughout the 20th century. Artificial ocean fertilization would help reverse this trend as a side effect.
While one ton of iron can theoretically stimulate algae growth to fix 80,000 tons of CO2, the actual sequestration efficiency is reduced by several factors. As noted above, the HSDC project was associated with a large increase in salmon population, which represents the transfer of carbon into the bodies of these fish. Some were caught and eaten, and others returned to the rivers of their birth to spawn. None of this carbon was sequestered, but the increased productivity of the salmon fishery and transfer of nutrients to Pacific coast forests are beneficial in themselves. Sequestration is dependent on downwelling currents separating phytoplankton from surface nutrient cycling. Because of this, choosing the right location to fertilize is of paramount importance. The Antarctic circumpolar current and areas of the Central Pacific with sustained downwelling are currently the leading candidates. Estimates of sequestration from recent iron fertilization experiments average about 20%, with the Southern Ocean giving the higher reported values.
While much work remains in sorting out the details, a quick calculation is possible at this point. Assuming a 20% near term sequestration efficiency in optimal sites, and accounting for inevitable inefficiencies in the distribution of iron, a sequestration of 10,000 tons of CO2 per ton of iron is reasonable. Global anthropogenic carbon emissions in 2017 were 41 gigatons. Were we to attempt to entirely offset these emissions with iron fertilization, 4.1 megatons of iron would be required. Global crude steel production in 2017 was about 1.7 gigatons, so this represents the diversion of roughly 0.2% of annual iron production, although using scrap and recycled iron would be preferable. Unfortunately, the algae would become limited by other nutrient deficiencies long before this point, there may not be enough downwelling areas in the oceans to remove such massive quantities of carbon from circulation, and global marine ecosystems would be heavily disrupted by such a massive ongoing fertilization campaign, though these challenges could no doubt be mitigated to some degree.
In a real world scenario including ongoing emissions reductions and limitations to fertilization efforts, 20 percent at most of this quantity of iron would be used—about 800,000 tons. This is equivalent to the capacity of 2 large oil tankers. If we assume the use of an easily soluble iron source, such as iron sulfate, this brings us up to 2.2 megatons, about 6 tankers per year. While this is economically non-trivial, it could be done for less than 10 billion dollars per year, comparing favorably with BECCS and DAC, which require human intervention at all stages of the sequestration process, and would cost at minimum several hundred billion in order to offset a significant fraction of current emissions.
Oceanic iron fertilization actually offers a very attractive and practical method to help solve the problem of global warming, assuming it is deployed alongside other negative emissions technologies and at least some degree of emissions reduction. There is no need to use only one method to the exclusion of all others.
Humans Now Control The Carbon Cycle
Examining the prehistorical natural analogues is vital to predicting the future consequences of different geoengineering methods. For example, the closest analogue to BECCS is the mass burial of terrestrial vegetation in the Carboniferous. Aerosol induced global dimming mimics the effects of large volcanic eruptions without the associated CO2 injection. DAC is a form of accelerated weathering. Iron fertilization has analogues in both the Azolla event and more directly in the addition of iron rich wind borne dust to the oceans during recent glacial periods.
While we don’t yet know everything, and we will continue to learn more about the costs, efficacy, and other effects of the different methods, oceanic iron fertilization appears very attractive. It is more economically efficient compared to BECCS and DAC, has none of the Faustian instability of aerosol injection, and has the significant benefit of increasing fisheries and oceanic ecosystem productivity.
What is clear is that a focus on emissions reductions alone is not going to work. Projecting climate impacts in terms of arbitrarily defined residence times of CO2 in the atmosphere ignores the fact that ocean dissolution is not an acceptable long term means of removing CO2, that natural sequestration is not a process occurring independently from human activity at a constant rate, and that even optimistic emissions reduction scenarios may throw the global climate into a period of severe instability.
Whatever our intentions, and independently of our fossil fuel emissions, humans now exert a dominating influence on the carbon cycle. We appropriate roughly 30% of the primary production of the terrestrial biosphere to grow food and produce raw materials such as wood, paper, and textiles. We cannot expect the biosphere to continue to function as though 7.7 billion humans are not present, simply because we reduce or eliminate fossil fuel use.
Unlike the many previous organisms which have caused global disruptions in climate simply by doing too well, we have the ability to intervene deliberately to mitigate the worst environmental consequences of our sudden dominance, and even stabilize and optimize the global climate system and carbon cycle to our liking.
This entails taking an active geoengineering approach where we stop acting as if the climate system is both beyond human control and too sacred to affect, and instead admit ourselves direct control, responsibility, and husbandry over the Earth. This transition from treating the Earth’s climate and ecological systems as wild and beyond the human order, to treating them as gardened integral parts of the human order, would massively impact how we see ourselves. New areas of opportunity would open up with the rise of such technologies.
For example, oceanic iron fertilization opens the door to fertilization and productivity management of ecosystems in general. What if we could turn deserts into productive pastures, turn barren areas into rainforests, boost the productivity and diversity of existing ecosystems, and have enough sustainable wild fish to feed everybody? What if we could choose to manage the earth to massively increase gross biological productivity using fertilization and careful control of the carbon cycle towards a massively more fertile and prosperous planetary ecosystem? These things are not immediately possible, and there will no doubt be pitfalls along the way; however, once we take the plunge into geoengineering, such possibilities start to seem almost inevitable.
On the other hand, we could also continue to stumble blindly toward extremely unpleasant climate disasters while desperately trying to minimize our impact, and descending into accusatory but practically useless political infighting. There is a clear choice here, and while geoengineering will require unprecedented global coordination and unity of purpose, it’s not even all that expensive. In the long term it is our only tenable option, and the opportunities are endless.
Patrick Mellor works to address the problem of CO2 accumulation in the atmosphere from a paleobiologically informed perspective. He is a co-founder of the company Living Carbon, and founder of the nonprofit Carbocene Industries. You can follow him at @resinoptes.bsky.social.