It’s Time to Build the Exoplanet Telescope
Decreasing cost to orbit makes new space structures viable. A transformative option is a telescope capable of seeing distant planets up close.
This article by Casey Handmer was published on Palladium Magazine on October 18, 2024. It will feature in PALLADIUM 17: Universal Man, shipping March 2025. Subscribe to the print magazine now to receive your copy!
With the recent SpaceX Starship orbital flight tests, it is time to commit to building the largest physically possible space telescope. Such a telescope would peer deeper into the universe than any before it, answering fundamental questions: are we alone? What do Earth-like exoplanets around other stars look like? How did we get here? What weird stuff awaits discovery? Where is the limit on human ambition to know what is in our universe? The Monster Scope answers these questions. Monster, because of its enormous scale, grotesque in its ambition. Monster, from the Latin root meaning a revealed thing. And monster, because through it we may be able to study not just the rocks and land masses but possibly lifeforms, both monstrous and marvelous, on distant planets.
When we look up into the night sky, we see thousands of stars. Most of them, visible to our weak and poorly-evolved eyes, are either exceptionally close or exceptionally bright. Along with the starlight that passes each moment through our corneas onto our retinas, its brother and sister photons splash uselessly onto the skin of our face, the ground around our feet, and the rest of the entire planet.
A telescope gathers this wasted light and corrals it into exquisitely sensitive instruments, extracting more of the ambient information that otherwise flows unseen and unstudied around us. The larger the telescope, the smaller and fainter the things it can see. Our pupils are but a few millimeters across, while the James Webb Space Telescope (JWST), one thousand times larger, can see objects millions of times fainter. For telescopes, a simple rule applies: the bigger, the better!
Since Galileo first used a military telescope to study the heavens, telescopes have followed a sort of Moore’s Law, doubling in size roughly every fifty years. There is no reason we should be limited by historical precedent. We are, collectively, much richer and more capable than our ancestors. We are curious, motivated, wealthy, and now, thanks to SpaceX, we have a really, really big rocket.
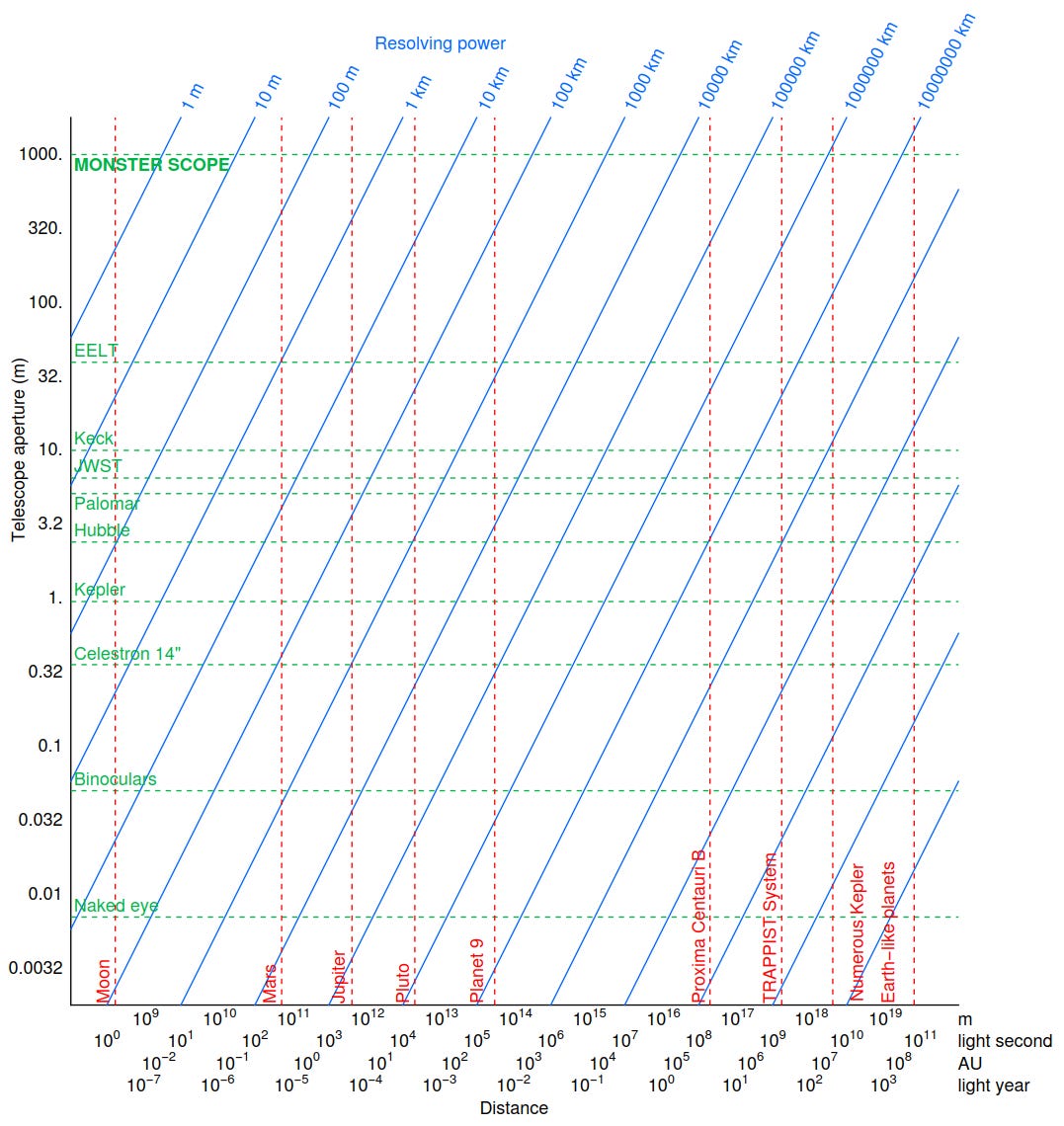
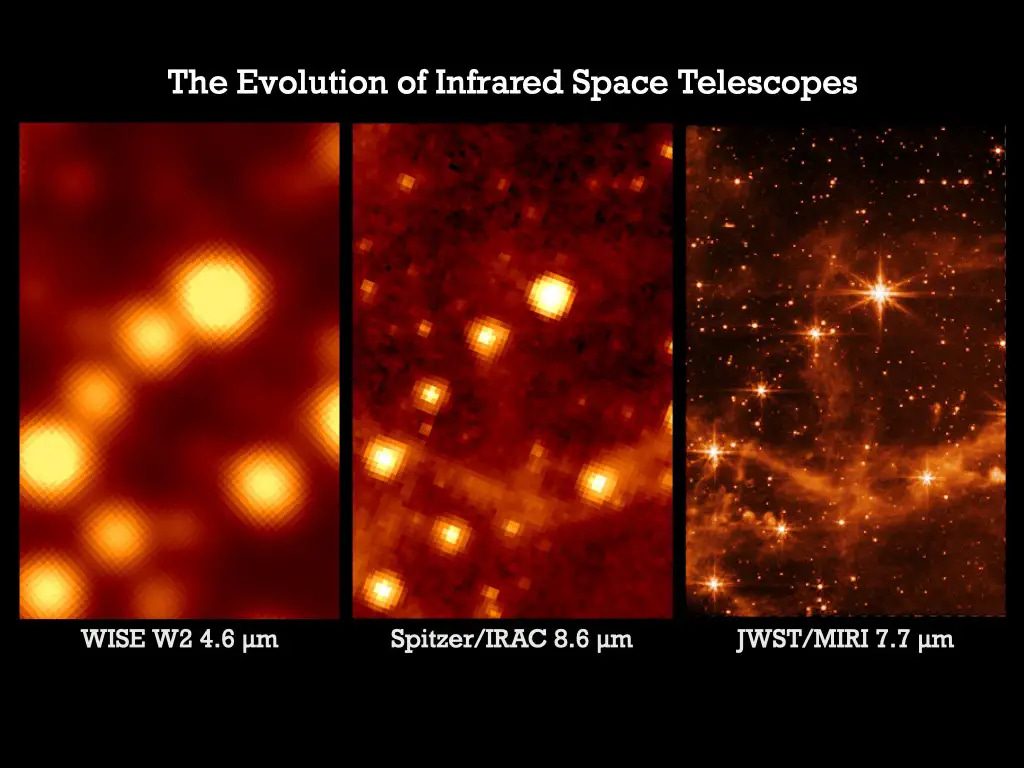
Historically, new space telescopes have been as expensive as they are infrequent. Despite the scientific success of the previous generation Big Four—Hubble, Compton, Chandra, and Spitzer—the JWST was so expensive and so delayed, reaching $9 billion over a $1 billion budget and fourteen years late, that future telescopes face an existential programmatic challenge. Programs get more expensive, more infrequent, and have ever-greater challenges maintaining institutional knowledge. Meanwhile, achingly slow development over years or decades drives up costs, further inhibiting future acceleration.
Look no further than the most recent Astrophysics and Astronomy Decadal Survey, published in November 2021. In it, the proposed “New Great Observatories” of four next-generation telescopes to be launched in the 2030s was considered too ambitious, in favor rather of a more expensive, more delayed, less ambitious, descoped all-in-one hybrid telescope to be launched in the 2050s. Two telescopes, LUVOIR and HabEx, focusing on different aspects of habitable exoplanets, have endured the indignity of an arranged marriage into the Habitable Worlds Observatory, cutting ambition, multiplying complexity, and extending timelines while compromising scientific utility. Current PhD students will be nearing retirement by the time these missions fly. We are literally up against the limits of the human lifespan as we scratch for crumbs against a backdrop of the greatest-ever levels of technical knowledge and wealth. There must be another way.
How does Starship help? Starship is designed to launch at least 150 metric tons to low Earth orbit at exceptionally low cost, with a launch cadence high enough to ultimately deliver over one million metric tons per year, roughly two thousand times the current global launch volume. This represents a transformation in human logistical capacity in space.
Why does this help telescopes? One of the reasons JWST was so expensive was that in addition to being exquisitely precise, robotically operated in a pitiless vacuum, and parts of it at cryogenically cold temperatures, it also had to be feather-light and capable of folding up like origami to fit within the Ariane V payload fairing i.e. nose cone. Starship has mass, volume, and launch capacity to spare, relaxing these engineering constraints and significantly simplifying design.
Starship can allow greatly reduced space development costs, provided that telescope and instrument designers internalize its capabilities and reallocate resources towards rapid iteration on a production-line style program. This can break the dismal spiraling cycle of programmatic bloat, slipping schedules, and eye-watering costs. JWST represents the pinnacle of what this sort of highly contingent, highly complex, extremely expensive development process can achieve. To progress further, it is time to take another approach.
A Self-Assembling and Ever-Growing Telescope in Space
The Starship internal fairing diameter is 8 meters. A Hubble-style telescope with a monolithic mirror could quite easily match JWST’s 6.5 m, and without any complex deployment procedure. Alternatively, a JWST-style folding process could extend the mirror up to 15 m, though it would definitely be cheaper simply to engineer a 16 m one-off Starship fairing and, once again, launch it as a monolithic instrument.
The largest of the next generation of ground-based observatories is the Extremely Large Telescope (ELT) at nearly 40 m, which pushes the limits of mechanical construction under Earth’s gravity. The mirror assembly can tilt up and down to follow a target as the Earth rotates, but as it does so, gravitational forces change throughout the structure, causing it to flex enough to disrupt the image. This problem, noticeable in the largest telescopes built more than a century ago, becomes exponentially more difficult at larger diameters. In addition, ground-based telescopes will probably never be able to image Earth-like planets around Sun-like stars because the contrast required exceeds the capability of adaptive optics to prevent the atmosphere from drowning the planet’s weak light in its sun’s glare.
Building a space telescope of 16 m in diameter and launching it on Starship is a fascinating idea, and we should absolutely do this ten or one hundred times. But Starship is so capable we should ask ourselves what the true physical limit is to our ability to build enormous telescopes. Assembling colossal mirrors in space sidesteps the gravity and atmosphere problems, so it is time to accept that the scope of our ambition exceeds the scale achievable on the surface of any planet, and use Starship to build transformational observatories in deep space: the Monster Scope has a mirror diameter of 1 kilometer, an unthinkably enormous span of glass enabling us to examine the features of nearby exoplanets with the same detail we can naturally see on the Moon.
This mirror is self-assembled in space from thousands of 8 m hexagonal segments, each an independent free-flying satellite and derived from the Starlink satellite design to keep costs and mass low. A self-assembling space mirror has been studied in detail with the AAReST concept, but the Monster Scope takes it to its logical extreme. These segments are stacked in the Starship fairing and launched by the dozen into deep space, for example Earth-Sun L2 Lagrange point—positions in space where the gravitational pull of the Sun and Earth combine such that objects in that region have the same orbital period as Earth—the same gravitational island used by JWST.
Once in position, they autonomously maneuver to the growing mirror, dock with adjacent segments and, once locked in, independently align their mirrors. A free-flying mirror position sensor (the blue cylinder in the diagram) flies at twice the focal length to provide the feedback needed to dynamically tune the shape of the mirror. This is a standard procedure for making mirrors and relies on interferometry, measurement of the interference of waves.
Some of the usual design constraints on focal length don’t apply in this case, as free-flying instruments in the imaging plane can be positioned beyond the radius of the mirror without obstructing the image. For our purposes, I imagine a configuration with an exceptionally long focal length optimized for resolving power.
The assembled and growing mirror forms a hyperbolic shape, which creates a larger focal plane covering perhaps 10 degrees of the sky. While the mirror can be turned, it will primarily be set up facing a particular set of chosen targets. Nearly all of the nearly six thousand extrasolar planets known to us today were discovered by the 2009-2018 Kepler telescope mission, which itself had a 10 degree field of view. There are worse ideas than following up on the Kepler exoplanets with a mirror 700 times larger!
The light from thousands of stars comes into focus at the focal plane, which occurs in a thin disk roughly 500 km from the mirror and 100 km across. Between the stars there are also opportunities to image and study distant galaxies and perhaps the first generation of stars, which are yet to be directly observed. Dedicated free-flying instruments and their host spacecraft (the gray boxes in the diagram) can navigate within this region. This approach allows the independent design and parallel operation of both the mirror infrastructure and the sensor payloads, increasing access to valuable science.
While one free flying satellite and large adaptable mirror may be extremely expensive to produce, combining thousands allows the production process to be greatly improved. The learning rate describes a property of manufactured products where unit cost drops by a fixed percentage per doubling of output. For products of this nature, a learning rate of 30% is conservative until we encounter fundamental material cost limits.
The similarly-sized mirrors, cast in glass, for the Giant Magellan Telescope, cost $20 million each. Starlink satellites are on the order of $100,000. Power electronics for piezo-adaptive optics are also about this price. Putting it all together, let’s baseline the first mirror satellite at $50 million, not including development costs.
The full 1 km telescope will require about 20,000 mirrors. With a 30% learning rate, running the numbers gets us down to about $300,000 per mirror and a cumulative cost of about $10 billion. The same as JWST, only 150 times wider and 22,000 times more sensitive. But why stop there? The mirror’s shape and scale is adaptable. We can continue to grow this mirror, or build another like it to focus on a different target, as the program progresses.
Answering Questions Heretofore Unanswerable
Whenever we build a new, more powerful telescope, we discover new and strange and wonderful things. Things that have been there all along, but invisible to us with our puny eyes and primitive technology. There’s an old joke that the quality of the astronomer is proportional to the size of the telescope. Instead of wasting the careers of scientists, engineers, and technologists on three decades of dead-end paper studies that teach us nothing about our universe and dissipate what little practical space telescope knowledge we already have, we can raise the scope of our ambition and build an exquisite instrument at the limit of physics, capable of imaging alien planets as though they were as close as our Moon.
The Monster Scope is designed to resolve features on the surface of exoplanets. Thousands of known exoplanets could be directly imaged, their continents, mountain ranges, and river systems studied from many light years away. Seasonal variations in plants and animals, if any, will be visible. Spectroscopic analysis of hundreds of discrete surface features per planet will tell us more about their geology than we knew about even Mars just forty years ago.
While it is true that aspects of this vision are new technology and unprecedented in scale, let us also remember that the Starlink constellation was science fiction for 35 years and now has 6400 active satellites serving four million customers. Starship represents a huge step change in our ability to execute missions in space and we should grasp it with both hands!
Humans have stared into the night sky for ten thousand generations and wondered “are we alone?” “Are there other worlds out there we can live on?” “Can we understand general relativity well enough to enable interstellar travel?” The Starship-enabled Monster Scope may answer these questions within our lifetimes.
Casey Handmer is a physicist who has worked at NASA and Hyperloop. He is the founder and CEO of Terraform Industries. You can follow him at @CJHandmer.